Spacecraft Overview
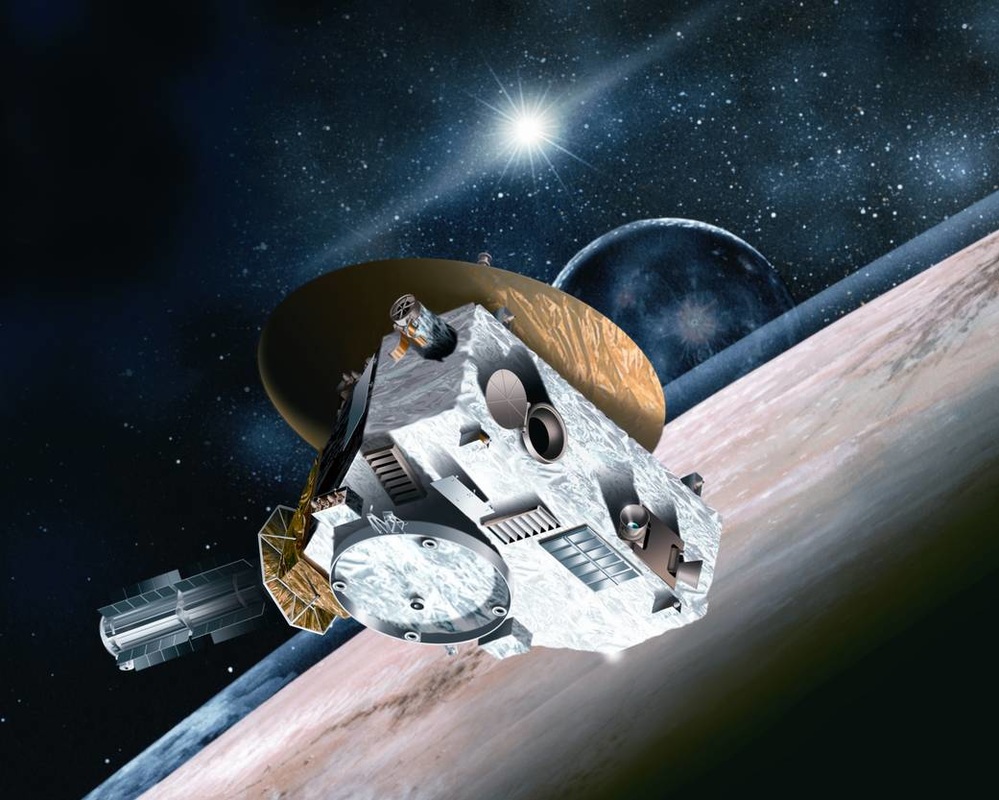
New Horizons is a Deep Space Exploration Mission operated by NASA, launched to study the Dwarf Planet Pluto and other icy objects in the Kuiper Belt.
Launched in 2006, New Horizons set the record for the highest launch speed of a human-made object, entering a trajectory to escape the solar system, set for a flyby of Jupiter before making the first-ever close flyby of Pluto in July 2015. The mission aims to gain data to help scientists understand the formation of the Pluto system, the Kuiper Belt and the transformation taking place in the early phase of the Solar System.
New Horizons was originally planned as a voyage to the only unexplored planet in the Solar System since Pluto was still a planet when the spacecraft was launched, later to be reclassified as a Dwarf Planet among its icy brothers in the Kuiper Belt, a zone extending from the orbit of Neptune out to about 50 Astronomical Units, filled with icy bodies.
Pluto’s reclassification as a Dwarf Planet will not lessen the scientific return from the New Horizons mission that will deliver the first-ever will resolved photos of Pluto and its largest moon Charon.
The understanding of the formation of the Pluto system and the Kuiper Belt will provide extremely valuable insights into the early transformation of the Solar System, filling in the gaps in knowledge from previous planetary missions.
Since Pluto and its companions build a third category of major objects in the solar system, alongside the rocky planets and the gas giants, the study of Pluto will deliver valuable data to understand where these bodies fit in.
Traveling over five billion Kilometers, New Horizons will deliver a package of seven instruments sufficiently close to Pluto to study its surface, its evolution, its atmosphere and interaction with the solar wind and many more aspects that will deliver completely new insights from a previously unknown world.
Spacecraft Overview
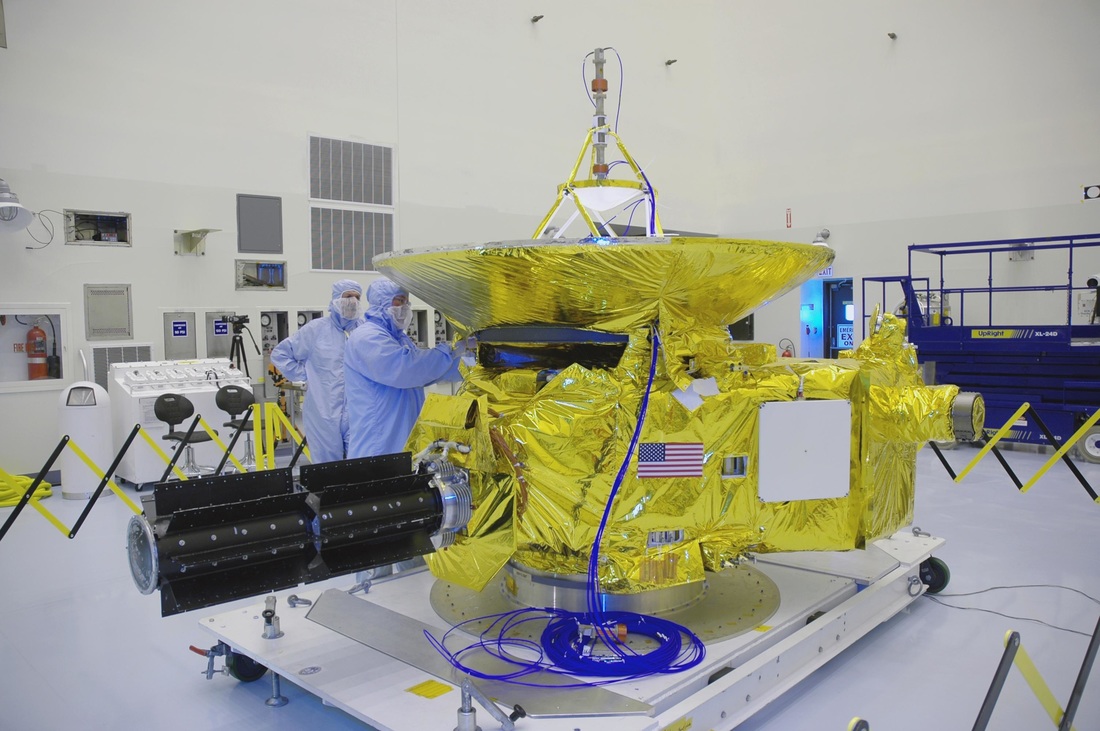
The New Horizons spacecraft has a total launch mass of 478.4 Kilograms and is 0.7 by 2.1 by 2.7 meters in size, often likened to a grand piano given its triangular shape and comparable size. The spacecraft consists of a Central Thrust Tube, a triangular spacecraft structure around the tube with a large High Gain Antenna Dish atop the spacecraft body and a radioisotope thermoelectric generator installed to the pointy end of the spacecraft bus for power generation, delivering barely enough power to run two 100-Watt light bulbs which is all the spacecraft systems and the science payload have to operate on. The spacecraft hosts seven instruments and purely relies on thrusters for attitude control.
New Horizons is part of NASA’s New Frontiers Program. The spacecraft was manufactured by the Applied Physics Laboratory of Johns Hopkins University while the Southwest Research Institute was responsible for the scientific instruments to which a number of participating institutions contributed.
Mechanical Subsystem
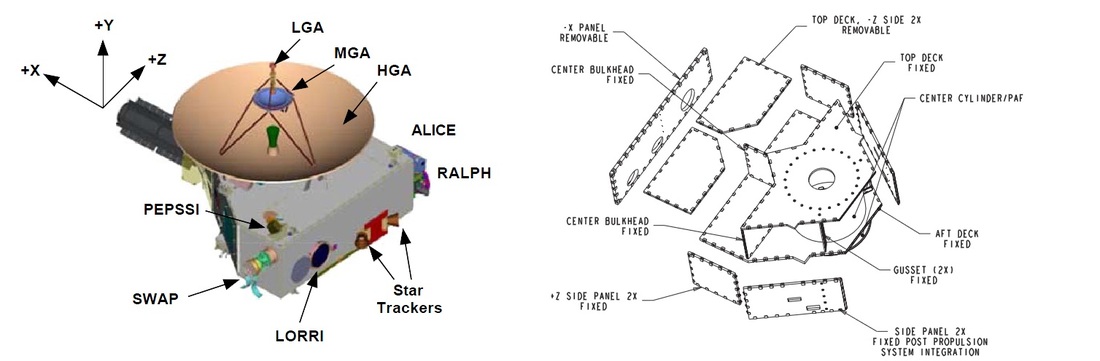
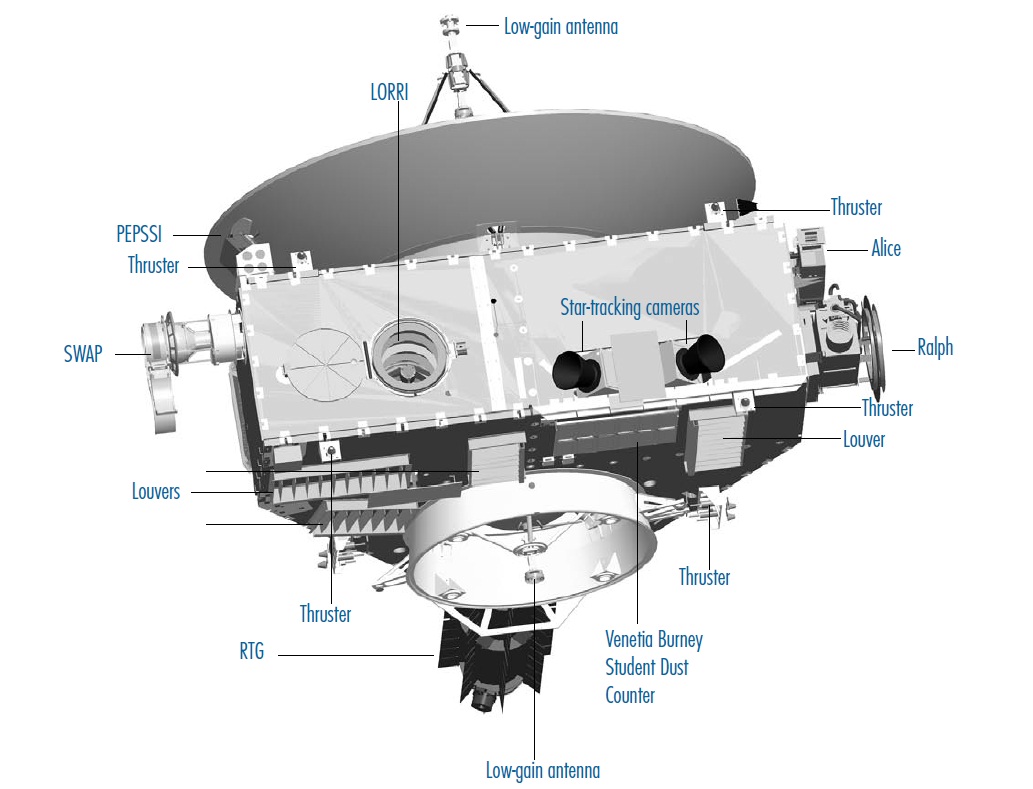
The New Horizons spacecraft consists of an aluminum central cylinder as the primary structural element of the spacecraft – forming the interface to the Atlas V launch vehicle and supporting larger vehicle components such as the propellant tank, the power source and a series of internal and external panels that provide mounting structures for the various instruments and systems.
The overall driver behind the structural design of the spacecraft was the compromise between stability and keeping the total mass low. Therefore, structural panels used on New Horizons use an aluminum honeycomb core with ultra-thin face sheets that are about as thick as two pieces of paper. Five side- and rear-facing decks are attached to the aft, top and main bulkhead decks. All these panels interface with the central cylinder and are used to support the various spacecraft components.
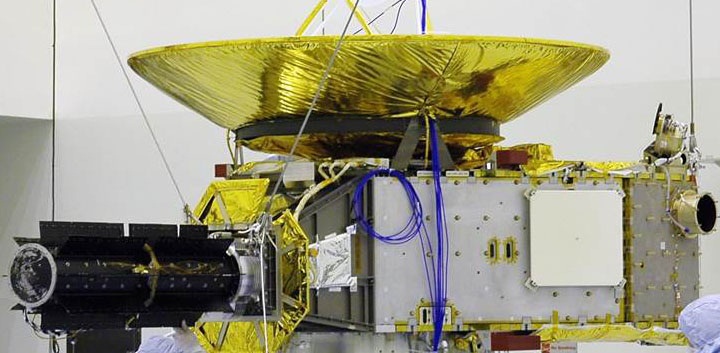
One important aspect of the New Horizons mission was to create a perfectly balanced spacecraft for proper spin stabilization during the long journey through the solar system. For that, the spacecraft underwent weighing and spin testing prior to launch and had a series of weights installed in strategic positions to fine-tune the spacecraft balance, properly placing the center of mass.
The overall design behind the New Horizons spacecraft called for the spacecraft principal moment of inertia to be on the same axis as the High Gain Antenna, the +Y axis of the spacecraft. Furthermore, the RTG was placed in the X-Z plane of the vehicle to increase the angular momentum of the craft and maximize the distance of the radiation source to the electronics and instruments. This spacecraft configuration provides a highly stable platform and eases the pointing of the craft to a specific direction, usually Earth, Pluto or any other science target.
Thermal Control System
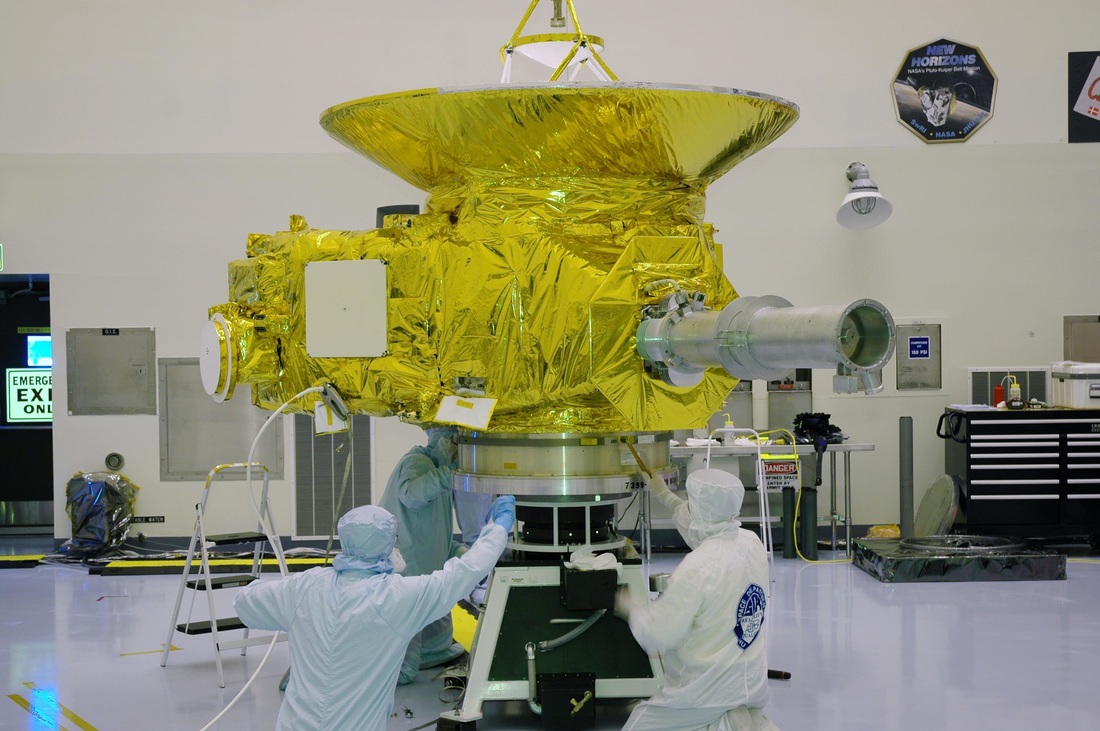
Thermal Control was a major consideration in the design of the New Horizons spacecraft since its mission takes it to the coldest places of the solar system and requires the spacecraft to keep warm over the course of its lengthy flight while also conserving power. The approach taken by New Horizons is to retain heat like a thermos bottle – New Horizons is already in the vacuum of space where no conductive and convective heat will be lost into space, leaving only the radiative dissipation of heat to be limited which is done by wrapping the entire spacecraft in a light-weight gold-colored blanket. The multilayer insulation blankets are designed to retain the thermal radiation emitted inside the spacecraft body by the various electronics to keep the spacecraft at an operating temperature between 10 and 30 degrees Celsius.
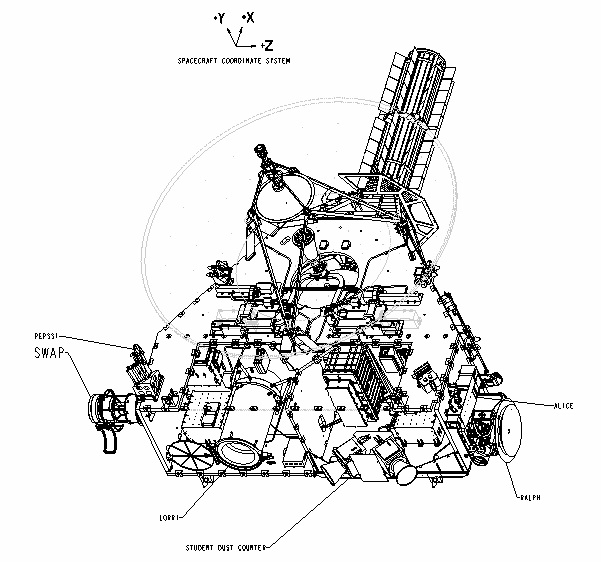
The location of the propellant tank has been chosen based on the consideration of the Center of Mass of the spacecraft, but also to shield the bulk of electronics from the radioisotope generator to keep their total dose as low as possible. Another factor was that the heat from the RTG can be usefully employed to keep the propellants from freezing.
New Horizon’s multilayer insulation is comprised of 18 layers of Dacron, a polyester fiber (a condensation polymer from ethylene glycol and terephthalic acid), sandwiched in aluminized Mylar plastic film and Kapton polyimide film. The Mylar and Kapton layers also provide protection of the internal spacecraft systems against micrometeoroids.
If the electronics of the craft are at the operational power draw of 150 Watts or above, heat generated by the various electronics components will be sufficient to keep the spacecraft at its operational temperature. New Horizons features a number of thermal sensors installed across the entire spacecraft and on components with the tightest thermal margins to alert the vehicle to dropping temperatures in which case heaters would be activated to maintain a safe thermal environment for all systems. The heater system is independent of the spacecraft computer and is capable of operating even in case of spacecraft safe modes.
To allow heat to escape into space when the spacecraft is too warm – which is the case when it is still close to Earth and the Sun – a series of louvers can be opened up to allow the radiative dissipation of heat from inside the spacecraft body. When in the outer solar system, these louvers will remain closed for most of the time.
Electrical Power System
New Horizons is the 27th NASA mission to employ a Radioisotope Thermoelectric Generator given the depth of space it will travel, far away from the sun where solar power generation would require extremely large solar arrays. RTGs are suitable power sources for Deep Space Missions since they deliver a predictable power over an extended period of time, permitting long mission durations to distant targets. RTGs deliver electricity through the conversion of thermal energy given off by the decay of a radioactive substance.
Spacecraft Block Diagram
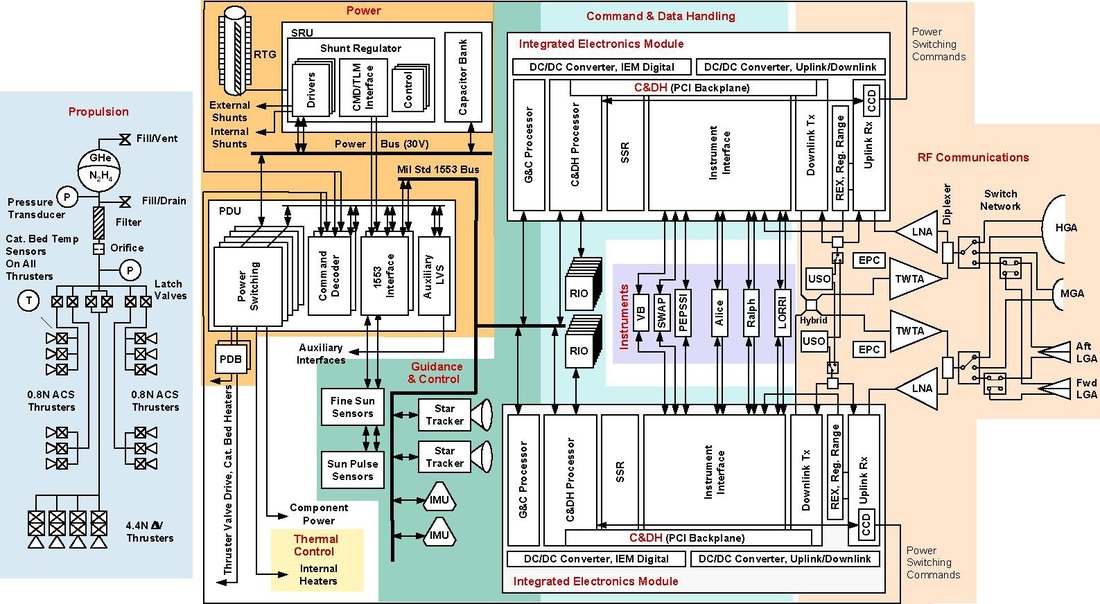
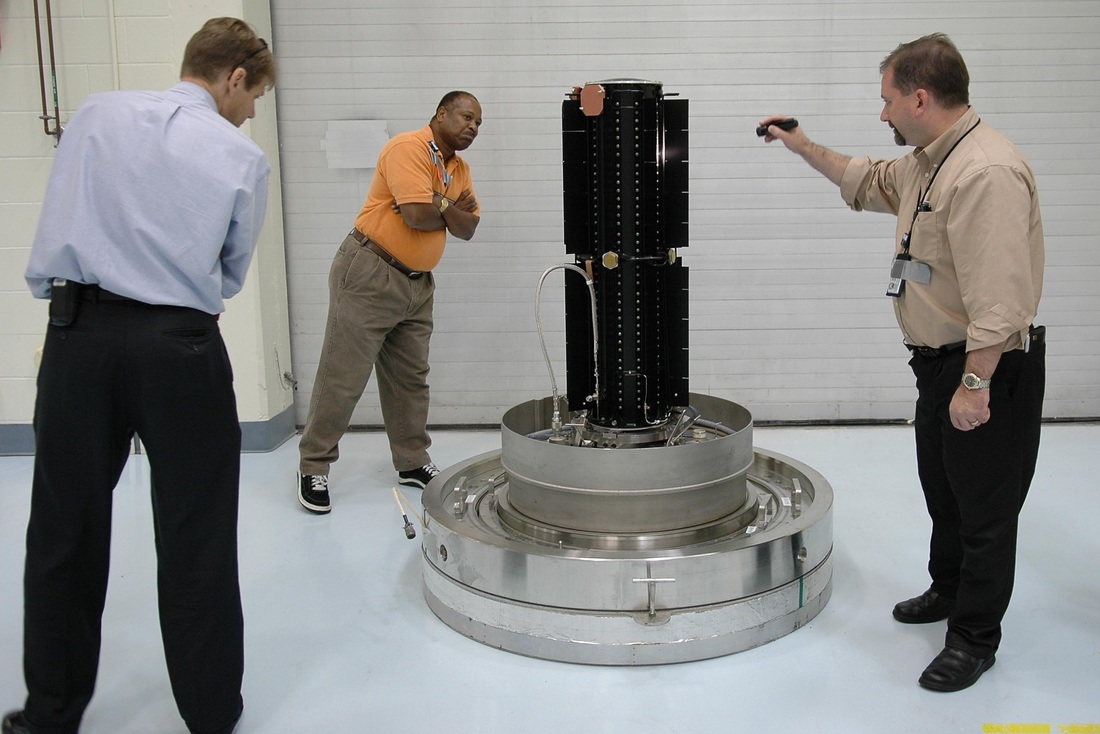
A number of missions have made use of this type of power generator including the Pioneer and Voyager deep-space probes, the Apollo lunar modules, the Galileo mission to Jupiter, the Curiosity rover on Mars and the Cassini mission to Saturn. It is from Cassini that New Horizons inherited its RTG, using a spare unit built for that mission. New Horizons uses a so called GPHS-RTG which stands for General Purpose Heat Source – Radioisotope Thermoelectric Generator.
The GPHS term refers to a standardized module designed for use in RTGs and SRGs (Stirling Radioisotope Generators) measuring 9.95 by 9.32 by 5.82 centimeters weighing no more than 1.44 Kilograms each and delivering 250 Watts of thermal energy at manufacture. Stacking of these modules can cater the output of thermal energy to the requirements of the mission. Each GPHS module contains four pellets consisting of a Plutonium mixture (Pu-236, 238, 239, 240, 241 & other isotopes from the Actinium group as well as chemically bound oxygen [oxides]). The pellets are surrounded by Iridium. The cladding provides sufficient alpha-particle shielding and and each pellet is nested in layers of carbon-based materials and placed within an aeroshell housing to comprise the complete GPHS module.
The GHPS-RTG contains 72 pellets that are grouped in four blocks to 18 units. Overall, the RTG weighs 57 Kilograms and measures 0.42 meters in diameter and 1.14 meters in length.
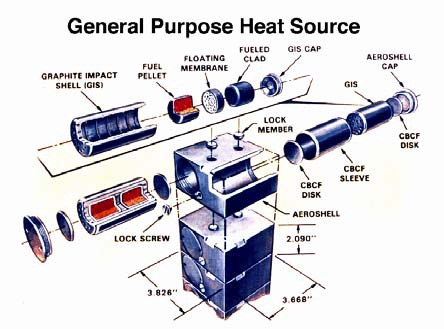
The total mass of plutonium dioxide within the RTG is 9.75 Kilograms. Structurally, the RTG is comprised of an RTG mounting flange holding it in place on the spacecraft, the General Purpose Heat Source with its GPHS support structure, a series of cooling tubes, thermocouples and foil insulation layers as well as a gas management assembly, a pressure relief device, cooling tubes and an Active Cooling System Manifold. The RTG is built with very high safety standards that guarantee that the unit containing the radioactive substance can survive a launch vehicle explosion and the subsequent re-entry and impact energy.
The RTG is attached to the New Horizons spacecraft on the opposite side of the central cylinder from the instruments. Titanium is primarily used in the RTG structure and its interface to provide a high degree of stiffness, low mass and low thermal conductivity. Electrical isolation within the interface is provided by multiple layers of non-conductive coatings applied to metallic plates located at the structure joints. The vehicle side uses an aluminum interface flange while the RTG-side features a titanium flange.
Thermocouples are installed in the walls of the container holding the fuel with the other end of the thermocouples connected to a heat sink so that heat flows through the thermocouples to the heat sink, generating electricity in the process. Thermocouples make use of the Seebeck Effect, converting thermal to electric energy. New Horizons employs silicon-germanium thermocouples that are referred to as unicouples due to the close physical proximity of the hot and cold junctions.
New Horizons uses Plutonium that was imported from Russia and mixed with leftover Plutonium from the Ulysses and Galileo missions, parts of which were already 20 years of age, reducing the overall thermal energy delivered by the RTG and thus preventing the system from delivering its specified power output of 285 Watts at the beginning of the mission.
All in all, the RTG has a total output of 3,948 Watts of thermal energy (at launch), but only 250W of electrical power can be delivered by the system, requiring the rest to be radiated into space. The excess heat is radiated into space using cooling tubes that transport heat to outer fins that use a black coating and maximize the active radiating area to prevent the components of the RTG from overheating.
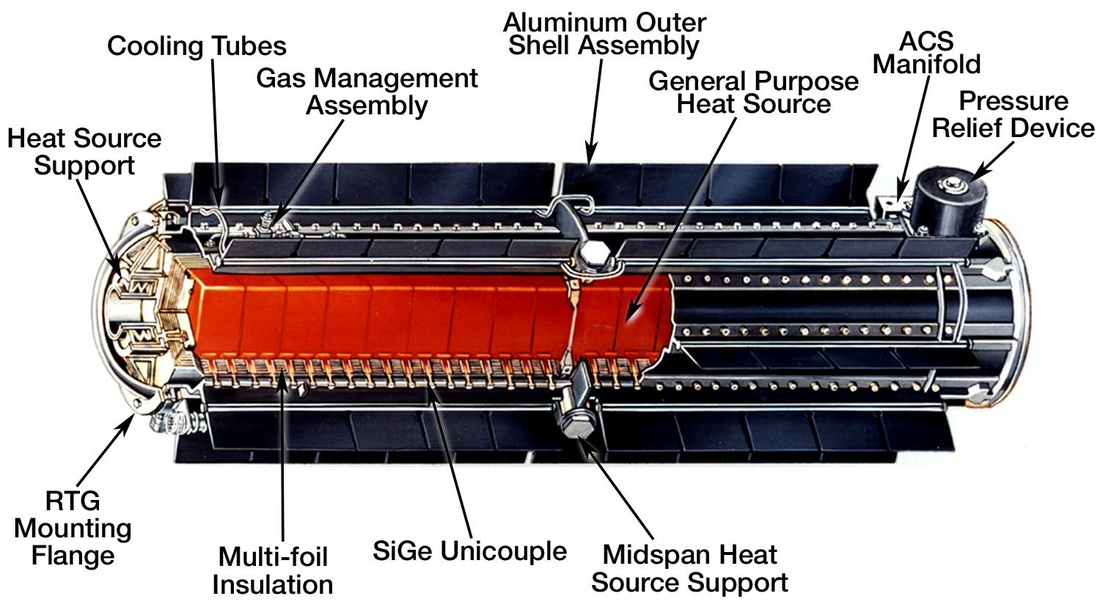
Before launch, the RTG is filled with an inert gas to prevent the unicouples from oxidizing. Once entering space the Pressure Relief Device vents the gas to allow the RTG to operate in a vacuum to increase its efficiency. Helium, released in the decay of Pu-238 to Uranium-234, is also vented overboard.
Power from the RTG is accepted by a Shunt Regulator Unit that consists of redundant Command/Telemetry Interfaces, Control Boards, Drivers and a Capacitor bank. The SRU maintains the power bus of the New Horizons spacecraft at a maximum voltage of 30V by dissipating the excess RTG power either in external resistive shunts, converting it again in thermal energy and radiating it into space, or in internal spacecraft heaters depending on the thermal status of the vehicle. The SRU includes three loop controllers using a majority voting system to control the 16 sequential analog shunts that maintain a consistent load on the RTG during spacecraft operational mode changes.
Each shunt can dissipate 19.5 Watts of electrical power and can be individually disabled if a fault is detected. The firs two shunts can be steered to internal heaters at half-power increments as controlled by relays in the SRU which maintain the configuration of the shunts.
The spacecraft bus voltage is set at 30.25V, slightly above the maximum power transfer level of the RTG to account for voltage drops when power is distributed to the individual loads. The capacitor bank has an electrical capacity of 33.6mF to dampen short-duration current surges when equipment comes on line.
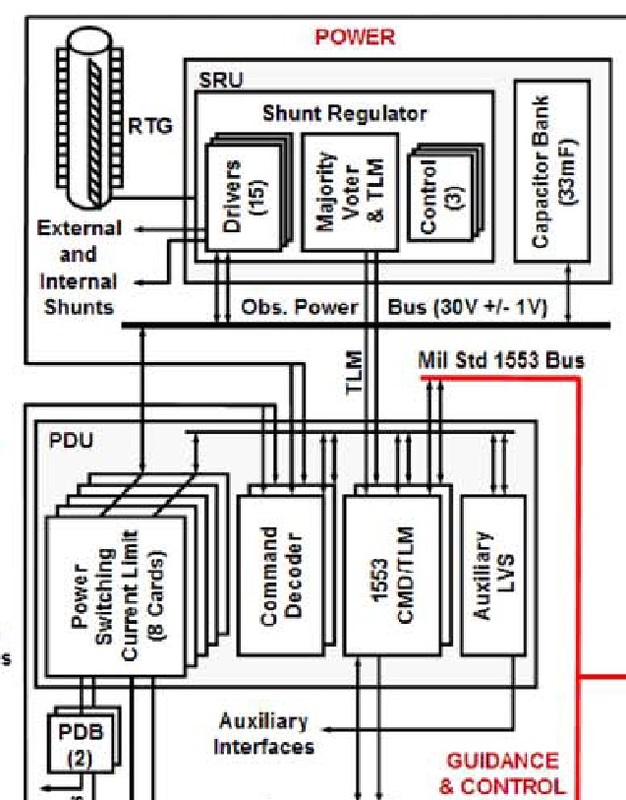
The next major element in the EPS downstream of the Shunt Regulator Unit is the Power Distribution Unit that hosts five Power Switching Units, two Command Decoders, and three data interfaces plus two Propulsion Diode Boxes. This design allows the PDU to provide two fully redundant solid-state power switching, pulsing and monitoring functions with hardware- and software-based fault protection and autonomy.
The PDU communicates with the spacecraft control system via two 1553 interfaces using redundant universal asynchronous receiver/transmitter (UART) serial links that pass critical commands and telemetry. PDU is in charge of delivering power to all loads of the spacecraft that are grouped in critical and non-essential loads. Critical loads are the Integrated Equipment Modules, the command receiver, Ultra-Stable Oscillators and Power Distribution Unit 1553 board – having their primary and redundant units powered at all times. To power off any of the redundant units, software and hardware-enabling would be needed, either through ground command or onboard fault-protection.
Load-shedding is employed to keep the power bus operating above a lower threshold. The PDU will start powering-off non-essential loads when sensing a bus voltage of 28.5 Volts and shut down additional non-critical loads at 27 Volts. Bus protection features provided by the PDU also include circuit breakers that have selectable levels for tight load monitoring. In case of problems, the circuit breakers can be opened and closed multiple times for power-cycling of loads. As a last-resort protection measures, loads can be permanently fused. The PDU also facilitates the sun sensor interface, spacecraft configuration relays and sensing of load telemetry (monitoring voltage and current) and temperature data from all power system components.
The Propulsion Diode Boxes, two for redundancy, build the power interface to the propulsion system components – actuating the thruster seat valves, catalyst bed heaters and propellant latch valves.
Overall, New Horizons’ power system can deliver 247 Watts of electrical power to users aboard the spacecraft at the start of the mission. Given the 87-year half-life of the primary constituent of the RTG, Plutonium-238, the output energy of the RTG will decrease over time, thus reducing the overall power availability. At the time of the Pluto encounter, New Horizons will have 202 Watts of power available while needing 176 Watts for spacecraft operations in full science mode which is a sufficient margin to ensure all operations can be completed. For an extended mission and a Kuiper-Belt Object flyby in 2021, around 182 Watts of power would be available, still permitting full science operations. The minimum power required by the spacecraft is on the order of 160 Watts for the operation of the central systems and the transmitter.
Navigation and Attitude Determination
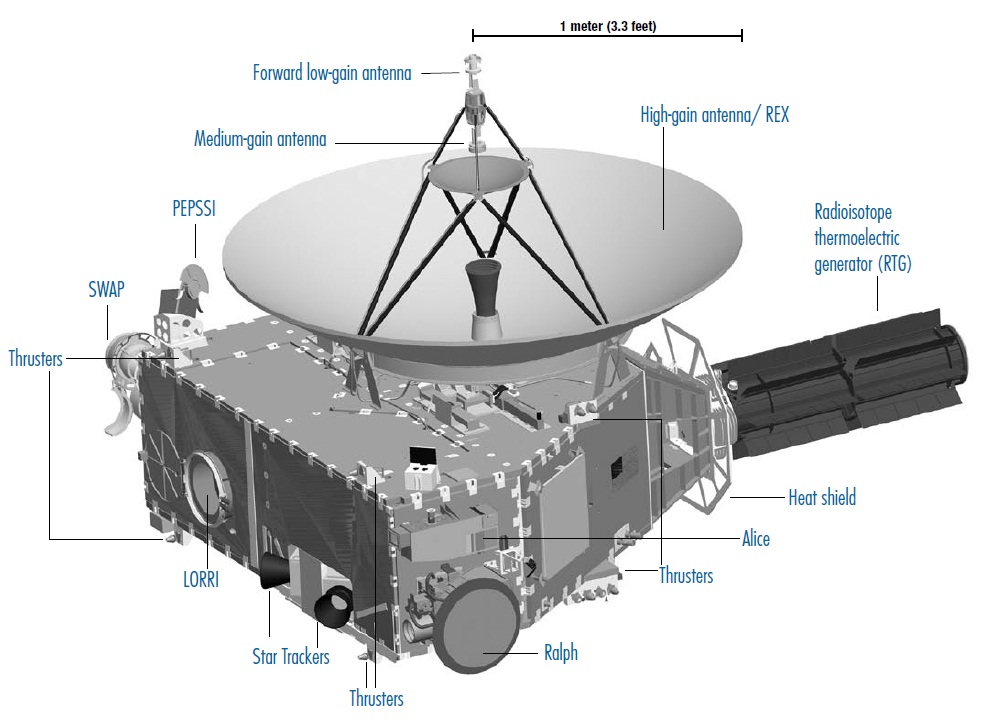
New Horizons uses a suite of sensors to determine its orientation in space and for the calculation of the solar, Earth and Pluto vectors which are the relevant pointing information for the different mission phases and for ensuring good communications with Earth.
The New Horizons spacecraft is outfitted with two Star Trackers, sun sensors and a dual-redundant Inertial Reference Platform to precisely determine its orientation in space and minimize body rates for scientific observations. Only one star tracker is active at any given time while the other provides redundancy in case of problems with the primary unit. The Star Trackers use Charged Coupled Device sensors to acquire ten images of the star-filled sky every second that are then compared with a catalog of 3000 known stars to determine the spacecraft attitude relative to these constellations and with that get a full three-axis orientation frame.
New Horizons combines Sun Pulse and Fine Sun Sensors. The Pulse Sensors provide an electrical pulse whenever the sun passes within their field of view to deliver data on spin-rate and help during attitude acquisition.
The redundant Fine Sun Sensors can not detect stars like the star trackers, but are built to detect the position of the sun with high precision which will be used in case of a spacecraft safe mode to point the antennas at Earth since the Earth and Sun vectors will be very similar once the spacecraft is at great distance to Earth.
Two Inertial Measurement Units incorporate ring-laser gyros to keep track of any accelerations occurring on any of the three axes to monitor body rates. The IMUs are also the primary sensor to measure achieved delta-v in Trajectory Correction Maneuvers. IMU data is delivered at a frequency of 100Hz.
The heart of the Attitude Determination System is the Guidance and Control Processor that hosts a Mongoose V processing unit clocked at 12 MHz. The 32-bit microprocessor is radiation hardened and was developed by Synova, Inc. with support from the Goddard Spaceflight Center. It first flew in 2000 and hosts 110,000 transistors. The processor has a direct-access memory of 16 Mebibytes.
Overall, the Attitude Determination System can determine the orientation of the spacecraft with an accuracy of +/-471 µrad in three-axis pointing mode. When the spacecraft is spinning, the attitude is also sensed at an accuracy of +/-471 µrad and the spin phase angle is known to within +/-5.3 mrad.
Propulsion & Attitude Control System
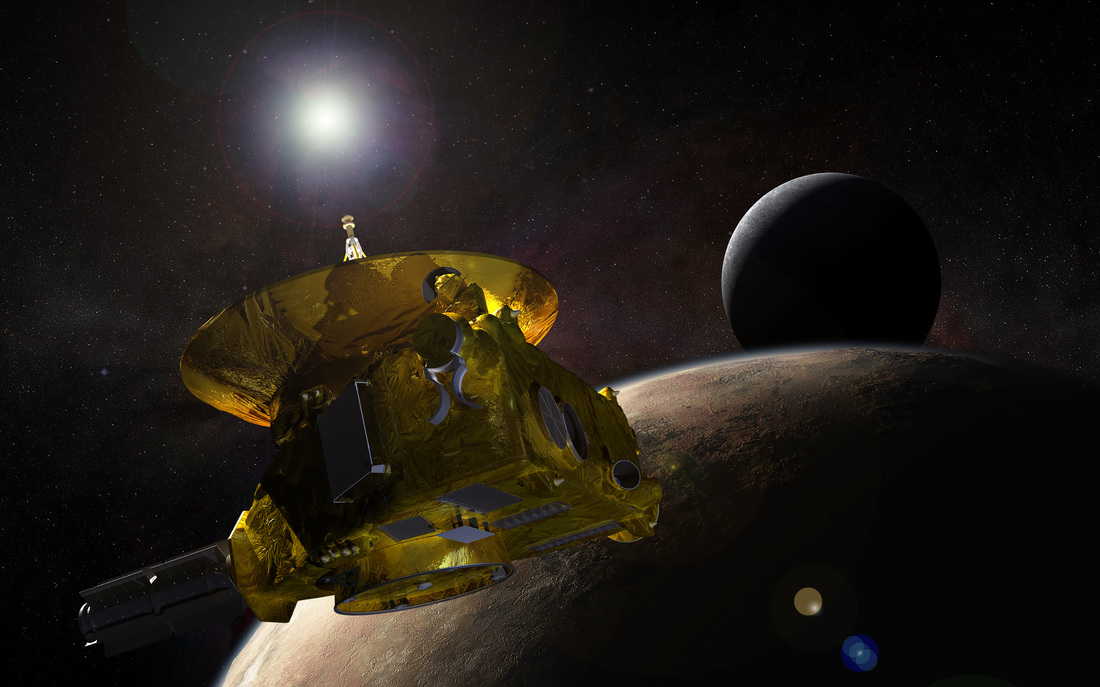
New Horizons purely relies on thrusters in propulsion and attitude control, there are no reaction wheels on the spacecraft. For most of its journey, the spacecraft will be spin-stabilized and only operate in three-axis control modes during testing, the Jupiter flyby, the Pluto approach & flyby, and other science observations as they arise during the mission.
The propulsion system uses monopropellant hydrazine thrusters that are fed from a single spherical titanium tank that resides within the central thrust tube of the spacecraft. The system operates in blow-down mode, meaning that the tank is pressurized pre-launch using high-pressure Helium-gas and is not re-pressurized in flight. The liquid and gas components are filled into the tank through dedicated Fill/Drain and Fill/Vent valves. Propellants fed from the tank are directed through a central propellant line, passing through a filter and a flow-control orifice before being directed to the various thruster banks.
Pressure transducers are mounted directly downstream of the tank and the orifice to deliver data needed for the calculation of the thruster output at the given inlet pressure which will go down as tank pressure drops over the course of the mission.
New Horizons hosts a total of 16 monopropellant thrusters arranged in five thruster banks. Four of these thrusters, making up a single bank, are used as the Main Propulsion System, each thruster delivering 4.4 Newtons of thrust to be able to conduct Trajectory Correction Maneuvers. The other 12 thrusters are arranged in four banks of three thrusters, each providing 0.8 Newtons of thrust. All the thrusters operate on the same principle, the catalytic decomposition of Hydrazine over a heated metal catalyst bed to generate combustion gases that are expelled at pressure to generate thrust. Catalyst bed temperature sensors are installed on each thruster to ensure proper heating of the catalyst material before thruster operation. Each thruster sports two heater elements, each drawing 2.2 Watts of power. (The heater circuit includes 16 switches with two heaters on different thrusters operated by a single switch in a measure that saves weight but also allows for very flexible operation of the thrusters.)
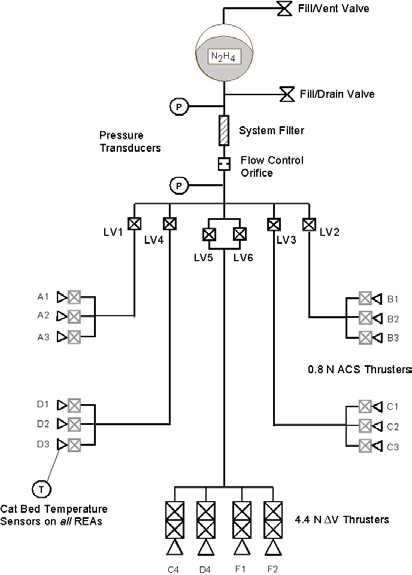
Each thruster bank is fed through a dedicated propellant line that can be isolated by latch valves while the main propulsion system features a dual-latch valve system for added redundancy. The 0.8N thrusters host a single seat valve while the main propulsion thrusters are outfitted with a dual-seat valve for redundancy.
The 4.4N thrusters are arranged in pairs, one is pointed to the –Y axis for delta-v maneuvers while the second pair provides thrust on the +Y axis and is angled 45° to the Y-Z plane to minimize plume impingement on the High Gain Antenna, reducing their overall propulsive effect, though they are still usable to provide redundancy and the ability to generate thrust in both spacecraft directions.
Each of the three 0.8N thrusters in a Reaction Control Assembly is pointed so that it can conduct coupled firings with one thruster from another set for the generation of torque on one axis for attitude and spin control. Uncoupled thruster firings will be employed for X-axis rates during science operations to comply with the maximum drift rates in these operational modes.
The single propellant tank of the New Horizons spacecraft has a capacity of 90 Kilograms and was filled at launch with 77 Kilograms of propellant and pressurant. It was never expected to fully load the propellant tank as the propellant mass loaded into the vehicle was dependent on the dry mass of the vehicle components as the total spacecraft mass could not be higher than the maximum payload capacity of Atlas V 551.
All in all, the mission requirement stated that 60 Kilograms of propellants had to be loaded into the spacecraft to complete the primary mission with some margin for unforeseen propulsive maneuvers. The mission hoped to add 9 Kilograms of propellant for an additional delta-v capability of 45m/s.
With 17 Kilograms of surplus propellant, the spacecraft has a total delta-v budget of 400m/s. The overall propellant usage plan for the mission included 22.3kg of propellant for Trajectory Correction Maneuvers, 29.3kg of attitude control and spin-management, a 17.5kg reserve for 91m/s and beyond that a 7.7kg surplus that could be used to generate 41m/s of delta-v. A change in the spacecraft spin rate of 5RPM will come at the cost of 0.125 Kilograms of propellant.
Attitude Control Modes
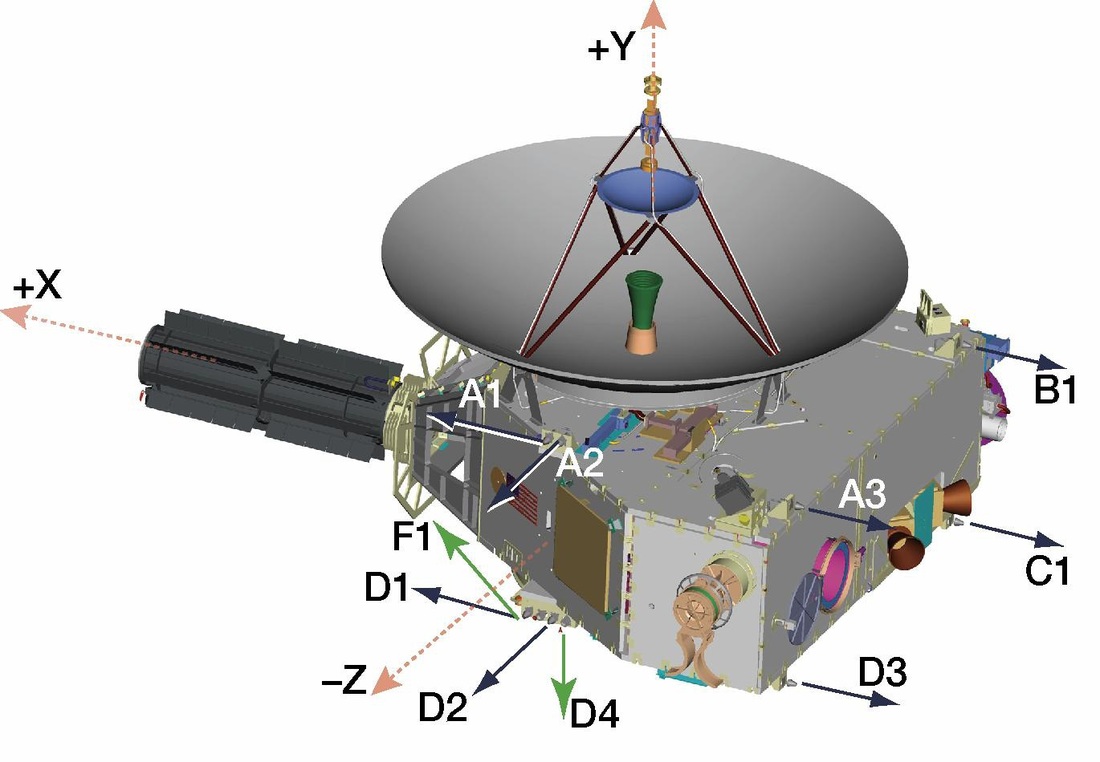
The New Horizons spacecraft can be operated in three principal attitude modes, 3-axis stabilized, Active Spin, and Passive Spin depending on the requirements of each phase of the mission. Trajectory Correction Maneuvers may be conducted in each of these modes, the choice driven by the magnitude of the maneuver. The various operational states of the spacecraft are chosen based on vehicle activity and other constraints such as the need to conserve power and propellant and the level of ground monitoring available for a given mission phase.
The most simple spacecraft mode for New Horizons is the Passive Spin Hibernation in which any attitude control is precluded, minimizing the electrical power demand and spacecraft monitoring as well as fuel consumption. This control mode is employed for the majority of the long cruise from Jupiter to Pluto. Another passive mode is the Passive Spin Normal setting that also precludes attitude control but does not limit other power demands making it suitable for use during portions of the cruise when spacecraft checks or other activities are to be performed.
The Active Spin Normal mode is used when an attitude maneuver is needed during a spinning phase of the mission. It is used to maintain the spin-rate of the spacecraft and precess the spacecraft to a new orientation.
In the three-axis mode, the spacecraft can be pointed in a particular direction or be rotated along a particular axis permitting the scanning and staring instruments to operate during the science phases of the mission. The 3-axis Normal state is normally used during instrument activities including commissioning, Jupiter science and Pluto approach. 3-axis Encounter mode is switched to active during the closest approach to Pluto. It is identical to the 3AN mode in the attitude control mode but reconfigures the Guidance and Control System of the spacecraft to prevent a spacecraft safe mode in case of problems with the vehicle to guarantee continued data acquisition during the most critical phase of the science observations. In the 3AE state, the autonomy of the spacecraft would be utilized to correct the fault while keeping the spacecraft pointed for observations.
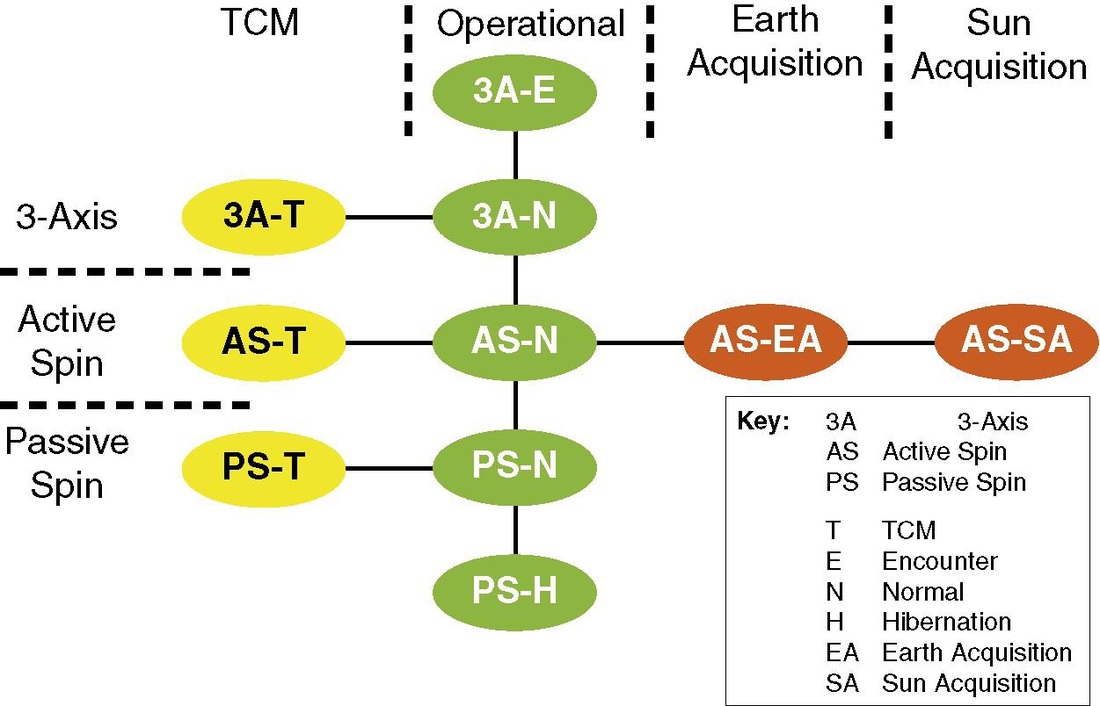
Safe mode is available in any attitude mode, except 3AE, and triggers one of two safe mode chains, pointing the spacecraft to the Sun or Earth, depending on its distance. The Earth Acquisition Go-Safe chain transitions the spacecraft in Active Spin Earth Acquisition and switches the communications system to the emergency uplink and downlink rates.
The Sun Acquisition Go-Safe is the last resort for New Horizons and is exercised only when communications from the ground can not be established in Earth Acquisition Mode for example due to corrupted onboard ephemeris, failed star trackers, or prolonged command loss. The Sun Acquisition Safe Mode only requires the sun sensors providing the guidepost for spacecraft pointing. In this mode, the spacecraft would send a red-beacon tone to alert operators on Earth of a major problem with the spacecraft.
Overall, the spacecraft attitude control system can keep the spacecraft body rates to within 24 µrad when in three-axis modes (34 µrad during spin-stabilization).
For science data acquisition, there are two basic modes of operation – keeping the spacecraft as still as possible to permit the staring sensors to operate, or controlling the rate along one spacecraft axis sufficiently precise to allow the scanning sensors to sweep out their required field of view and conduct time delay integration. The staring sensors require the spacecraft to reduce its rotation to less than one image pixel per exposure.
The thrusters are operated in pulse mode with a pulse length of 5 milliseconds to achieve the desired control precision with the Inertial Measurement Units used as the fine rate control sensors. The characterization of all thrusters and their specific performances and potential deviations from the norm was completed as part of spacecraft commission for accurate prediction of maneuvers that are required for attitude and spin rate changes.
Command and Data Handling
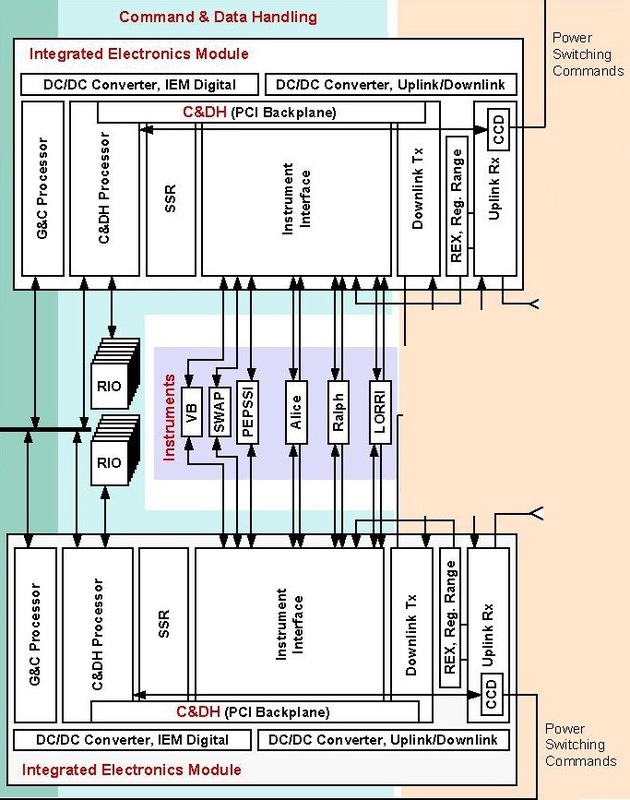
The Command and Data Handling functions completed aboard the New Horizons spacecraft include command management, science and engineering data management, timekeeping, data storage, data transmission and autonomous system recovery and safing. These tasks are implemented with the resources within two redundant Integrated Electronics Modules that are identical in architecture and each contain a Command and Data Handling Processing card, a Solid State Recorder Card, an Instrument Interface Card, a Critical Command Decoder Card for Uplink, a Downlink Data Formatter and Downlink Card. The various cards of the IEM are connected by a Peripheral Component Interconnect (PCI) Backplane.
A series of Remote Input/Output units deliver the various data streams from the spacecraft to the IEMs. These data streams include health monitoring parameters such as voltage and temperature measurements from the various components of the vehicle. The RIOs communicate with the Command and Data Handling system via an integrated circuit (I²C) data bus. An 1553 data bus is used between the two IEMs for redundancy and cross strapping as well as the exchange of commands and data between the Guidance and Control components and their appropriate processors.
The Processing Units of each IEM comprise a Mongoose V processor that is clocked at 12 MHz can handle 15 MIPS (Million Instructions per Second). The processor has a 2K data cache memory and 4K instructions cache. It can transfer data at up to 480kbit/s and is connected to the experiment payloads via analog RS-422 links for command transmissions. 768Kbyte of memory are available to macro instructions (detailed below).
In the normal mode of operation, one of the IEMs and all its components is fully powered and acting as primary spacecraft controller while the other unit is in a standby mode.
During the long quiescent cruise from Jupiter to Pluto, the standby unit is powered off altogether. Since the IEM is in charge of all spacecraft monitoring tasks, fault-protection and autonomy, a second system had to be implemented to monitor the performance of the C&DH system. This task is accomplished within the Power Distribution Unit’s data system and the Guidance and Control Processor that are both monitoring a heartbeat pulse sent from the C&DH system at one pulse per second. In case this PPS signal is not present for 180 seconds, the redundant C&DH string will be powered-on and assume command as the primary bus controller. This change would also alert teams on the ground via an emergency signal.
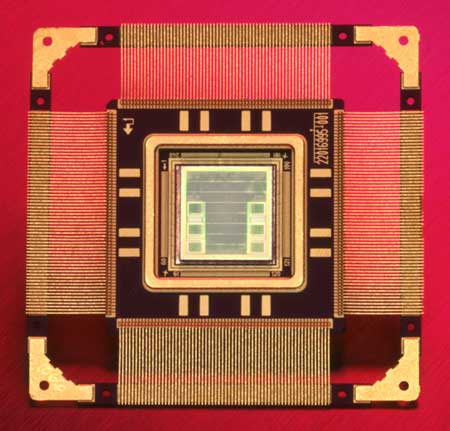
Commands from Earth are received by the antennas of the spacecraft and then directed to the RF Uplink Card within the IEM. A critical command decoder on the uplink card is in charge of receiving and executing commands without action of the rest of the C&DH system such as processor re-starts, switching of C&DH controllers and other power-switching commands. All other commands are delivered to the C&DH system via CCSDS (Consultative Committee for Space Data Systems) telecommand transfer frames. The telecommand packets are then extracted by the software and transferred to spacecraft subsystems for execution or to the C&DH processor itself. Commands are either tagged to be executed in real time or include time-tags for later use. These commands intended to be executed at a later time are known as command macros. Macros can be simple on/off commands for given systems or consist of a string of switching actions and information for use by a designated subsystem. Each C&DH subsystem has 0.75MB of storage for macros and New Horizons already launched with 132 macros on board to be accessed by the C&DH system as part of its autonomous authority.
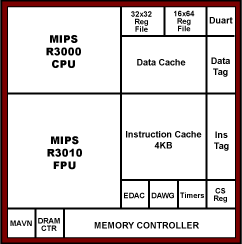
Time-tagging is used for the majority of commands executed as part of science sequences at Jupiter and Pluto. New Horizons can store up to 512 time-tagged rules.
Autonomy is a major component of the Command and Data Handling system given the large distance the spacecraft will travel and the associated delay in two-way communications. Therefore, to preserve consumables and science observations, it is in the mission’s best interest to implement autonomous features to keep the spacecraft in an operational state by correcting faults on its own instead of jumping into a safe mode straight away which would be a common fault response of a spacecraft in Earth orbit.
Basic elements of the onboard fault detection system reside within the redundant elements of the Power Distribution Unit which monitor the C&DH bus traffic and can automatically command a switch of processors if the normal C&DH activity ceases for some reason. The more advanced elements of the onboard autonomy system are implemented by software running on the bus-controlling C&DH processor.
The C&DH software completes a real-time analysis of the telemetry delivered by all subsystems and takes action in case of an off-nominal situation. Actions that can be taken by the C&DH system can either be the execution of a set of commands to correct the detected fault, the transmission of a beacon tone to alert mission operators of the off-nominal event, or place the spacecraft in one of two safe modes, Earth Acquisition Safe Mode and the Sun-Acquisition Go-Safe mode which would also be accompanied by the transmission of emergency signals to Earth.
The algorithm used to analyze onboard data includes specific limits for all parameters allowing the system to identify any out-of-spec parameters that are then monitored to track the persistence of the exceedance of the given limit. The persistence length varies from rule to rule to avoid the C&DH taking action based on noisy data or transient data as a result of commanded changes in spacecraft state. All processors implemented in other onboard systems are monitored via their Pulse Per Second heartbeats and re-set in case they get stuck in a state.
The autonomy system can also employ dynamic limits in which it compares the current state of a system with a set of vales recorded at a previous time appropriate to the current mode of spacecraft operation. This includes the propulsion system that is checked for propellant leaks by monitoring the propellant via sensor readings from pressure and temperature transducers that are input in the ideal gas law to compute a current value and compare it to a previous value obtained previously – any unexpected change would indicate a leak. When being launched, New Horizons has 126 rules it could access to autonomously determine the spacecraft state of health. Over the course of the mission, new rules can be added as teams learn about the peculiarities of the spacecraft and come up with more corrective measures that can be automatically implemented. A total of 512 autonomy rules can be stored on the spacecraft.
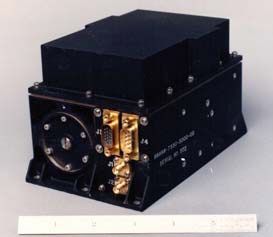
For the execution of time-tagged commands, it is of major importance that the spacecraft Mission Elapsed Time (MET) is accurately correlated to Universal Time since commands generated on the ground will use UT to plan all navigation, control and science operations. New Horizons uses two Ultra-Stable Oscillators as the onboard source of accurate 1 Pulse Per Second signals as the spacecraft time base. The C&DH system includes a carefully designed UT to MET clock register that achieves an onboard correlation of better than +/-4 seconds when the spacecraft is at Pluto (a 9-hour round trip time to Earth at the speed of light). Science instrument time correlation has to be accurate to +/-10 milliseconds.
Each of the two Integrated Electronics Modules includes an Instrument Interface that handles the two-way communications between the C&DH System and the instruments which includes the transmission of commands and MET from C&DH to the instrument, the collection of housekeeping and low-rate science data from the instrument, the reception and formatting of instrument high-rate data and IEM header data, the distribution of spacecraft time markers to the instruments, the processing and transfer of IEM analog telemetry from the RIOs.
Each instrument interface provides connections to both sides of each instrument’s redundant electronics thus ensuring proper cross-strapping between the instrument sides of the data handling system.
The Solid State Recorder hosted in each IEM consists of 64Gbits of non-volatile memory, organized in 16 addressable segments that correspond to 16 physical memory stacks on the SSR card. The Solid State Recorder can accept science data at up to 13Mbit/s. Data is recorded in a functional segment until it is full, prompting the SSR to switch to the next with no delay. Raw data is processed into compressed data frames and re-written in the SSR for later downlink. The SSR is erased on a segment-to-segment basis requiring a complete segment to be transmitted or compressed before it can be erased. New Horizons handles 51 different types of data from different housekeeping data formats to the different raw, low-rate, high-rate and compressed data types specific to each of the instruments.
Data written in the SSR can be accessed by Mission Elapsed Time or bookmarks that can be assigned to data gathered as part of a specific activity for easier access and downlink. Playback data can be accessed by MET, bookmark and data type.
Compression algorithms employed by New Horizons include lossless and lossy compression, both using CSSDS packet standards. Image data can be put through a subframing algorithm that isolates features displayed in images (up to eight frames per image) and then performs lossless compression of the frames that contain features, discarding empty image segments thus cutting useless data from the downlink.
Communications System
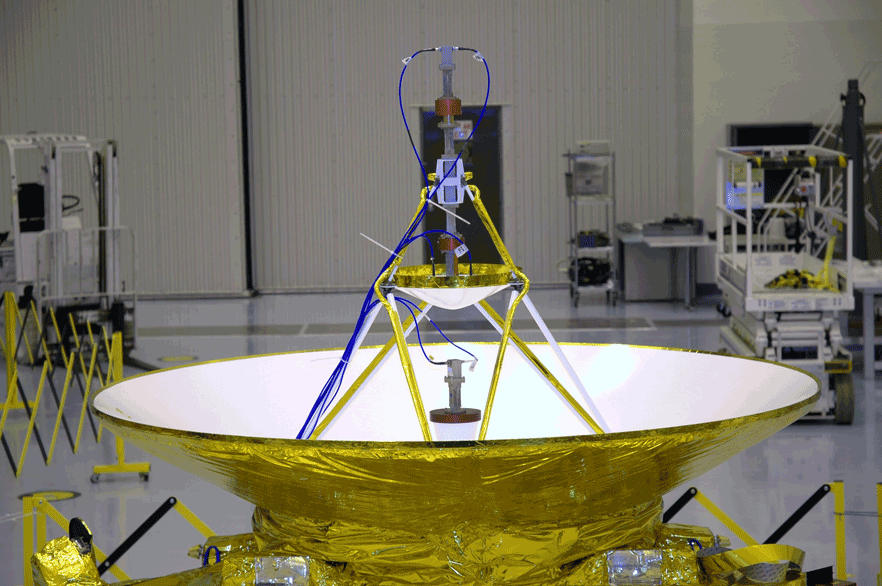
The Communications System of the New Horizons spacecraft provides the command uplink, telemetry and data downlink and essential elements of the REX instrument and radio navigation capabilities. It is designed to provide two-way communications with NASA’s Deep Space Network in both spinning and 3-axis stabilized attitudes at distances greater than 50 Astronomical Units.
The RF Telecomm System is comprised of an antenna assembly, RF switches, hybrid couplers, redundant amplifiers, redundant Ultrastable Oscillators and the uplink/downlink cards within the two Integrated Electronics Modules. New Horizons is equipped with directional antennas – a single High-Gain Antenna, and one Medium Gain Antenna, as well as two omni-directional Low Gain Antennas.
The forward antenna system is comprised of the High-Gain Antenna, the Medium Gain Antenna, and one of the Low Gain Antennas, all installed on the –Y panel of the spacecraft. The other Low Gain Antenna sits on the +Y side to achieve an omni-directional low-gain capability.
The High-Gain Antenna parabolic dish measures 2.1 meters in diameter while the Medium-Gain Antenna uses a 30-centimeter dish antenna. The HGA beam is only 0.3 degrees wide requiring the spacecraft to be pointed accurately at Earth while the Medium-Gain Antenna transmits a 4-degree beam for which it will be sufficient to have coarse Earth pointing or pointing the spacecraft to the sun since Earth, as seen from Pluto, will be within a small sector to the sun. The Low Gain Antennas can only function to a distance of around 1 AU, providing omni-directional communications for the initial mission phase and commissioning.
The High Gain Antenna was designed to be able to deliver downlink data at a rate of 600bit/s (per polarization) even at a distance of 36 AU for the playback of Pluto data. New Horizons will be able to receive commands up to a distance of 50AU or beyond.
RF Comm System Block Diagram
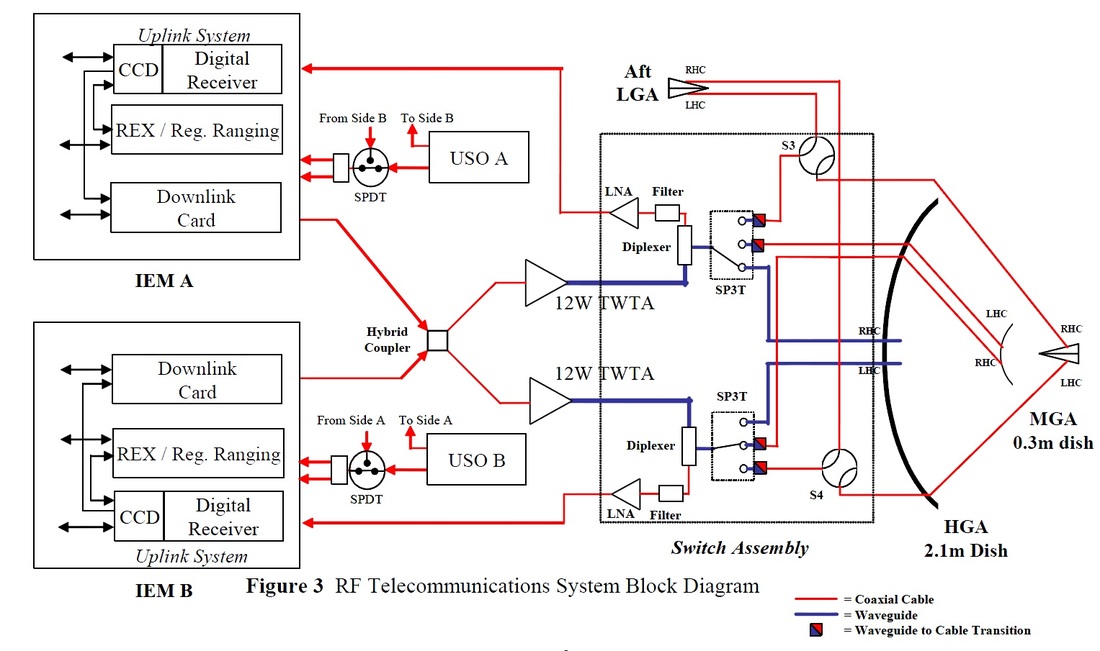
The overall architecture of the RF Communications System is fully redundant with two parts of each component and abundant cross-strapping between the two strings to allow failed components to be bypassed. Starting from the antennas, commands are directed through a network of switches to the Diplexer that separates the downlink frequency from the uplink signals, then to a Low Noise Amplifier and then to the Uplink Rx Card in one of the Integrated Electronics Modules.
Downlink data travels the opposite direction, starting out in the Downlink Transmitter Card within the IEM, then being routed through a hybrid switch that couples the two strings, then to a Traveling Wave-Tube Amplifier, through the Diplexer and the switches to the appropriate antenna. The Ultrastable Oscillators are directly cross strapped and interface with the downlink and uplink cards of the IEMs. Furthermore, a REX/Ranging card is directly connected to the Uplink Receiver and interfaces with the Instrument Interface for the acquisition of REX data and the C&DH processor for ranging operations.
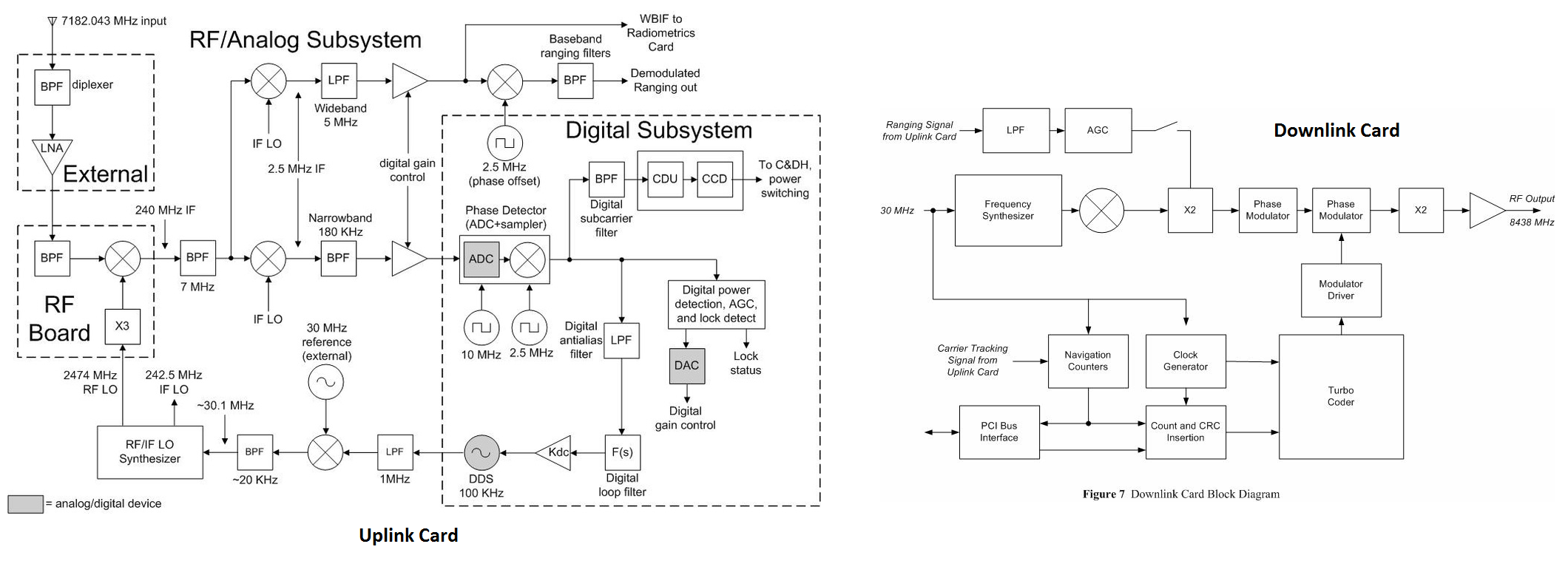
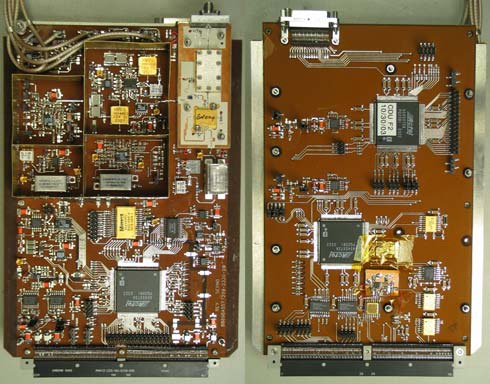
The Uplink Command Receiver on New Horizon uses innovative digital systems to drastically reduce the power requirement of the system to 4W per receiver. Previous systems required on the order of 12 Watts which, with two units that are usually active, means New Horizons is saving 16W of power – with a total power budget of less than 200W this can make a huge difference in the critical science capabilities of the spacecraft. The Uplink Card also provides command decoding, ranging tone demodulation, X-Band carrier tracking and a regenerative ranging subsystem. The non-coherent Doppler tracking and ranging system developed by APL is also largely implemented on the Uplink Card, capable of Doppler velocity measurements better than 0.1mm/s.
The Regenerative Ranging Card, also supporting the REX instrument, facilitates a new ranging capability to provide more accuracy and easier operation as the traditional method of sending a phase-modulated tone to the spacecraft that is received and immediately re-transmitted to determine the two-way light time and thus the distance to the spacecraft. However, this method is vulnerable to noise in the ranging modulation signal on the spacecraft which is amplified and retransmitted back to Earth. One-tone ranging can be supported by New Horizons, but the new Regenerative Ranging can limit turnaround uplink noise.
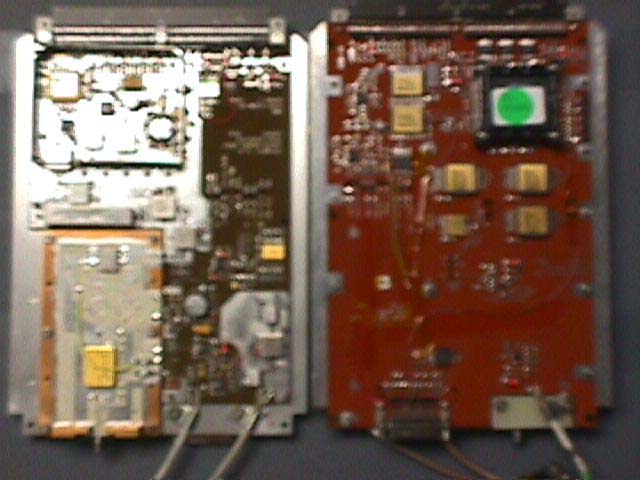
The system uses a delay-locked-loop that generates an onboard copy of the ranging signal and adjusts the timing of the onboard signal to align it with the received signal. The signal transmitted to the ground is free of uplink noise and reduces the primary error source contribution. This method could permit the determination of spacecraft range to within ten meters at a distance of 50 AU or beyond.
The Ultrastable Oscillators are a critical component of the spacecraft since they deliver the precision reference frequency at 30MHz used for the uplink, downlink and radio science experiment as well as the keeping of precise onboard timing solutions. At its heart, each USO has an ovenized crystal oscillator with a short-term frequency stability of 2*10^-13 at the 10-second interval.
Regenerative Ranging
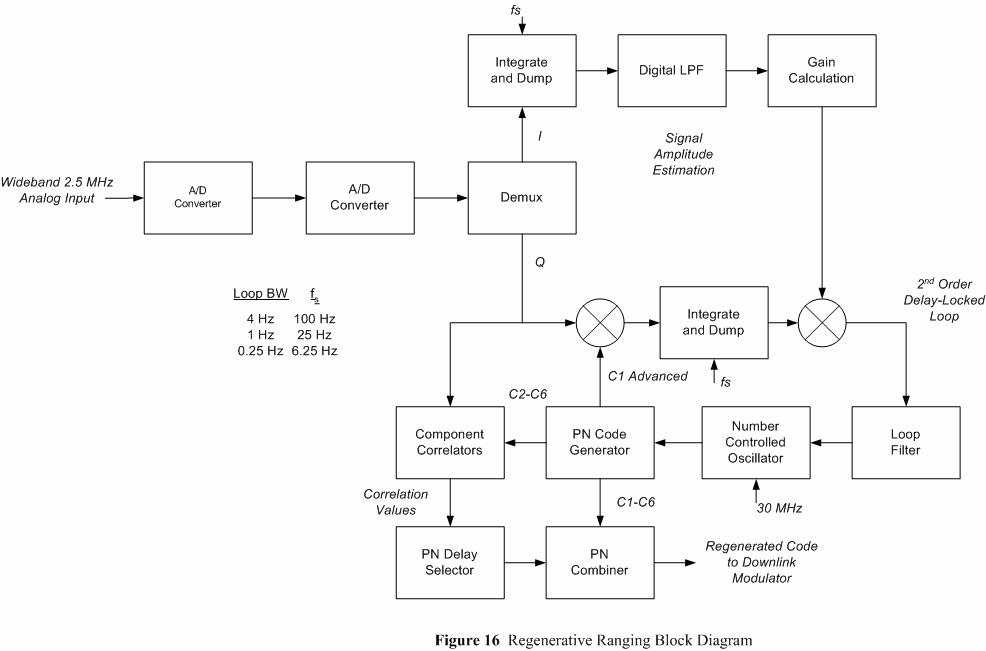
The downlink system generates its signals within the exciter of the Downlink Card and encodes frame data from the C&DH system into CCSDS-compliant blocks for amplification. Also inserted into the data are Doppler Tracking Blocks for the noncoherent tracking. Additionally, the system is in charge of forming and transmitting beacon tones during hibernation. The exciter output is coupled to the TWTA RF input by a hybrid switch so that either downlink card can be connected to either of the two TWTAs.
The switch network can allow signals from both TWTAs to be transmitted simultaneously through the High Gain Antenna to (almost) double the downlink rate to 1200bit/s, but only if spacecraft power permits. In this case, one signal would be right-hand polarized while the other system delivers a left-hand polarized signal. The combined signal can be received by a DSN station and enhance the received signal-to-noise ratio and so increase the data rate by 1.9 times above that using only a single TWTA.
The design of the RF system guarantees that the entire Pluto data set of 5Gbits (after compression) can be downlinked in 172 days, assuming one 8-hour comm pass per day using a 70-meter DSN station. If power allows both TWTAs to operate for all passes, the downlink time would be reduced to 88 days.
New Horizons Instruments
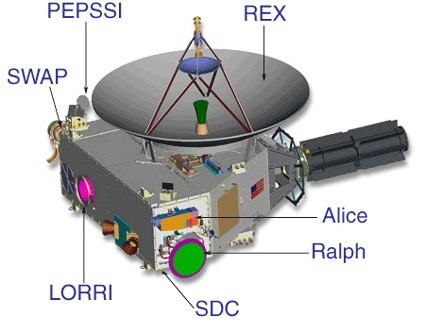
The New Horizons spacecraft is outfitted with six primary science instruments and one student-operated payload, driven by the power and mass requirements of the mission.
The instruments are installed fixed to the spacecraft structure, requiring the craft itself to change its orientation for pointing of the instruments. New Horizons is equipped with instruments covering optical imaging, spectroscopy in multiple bands, as well as plasma, particle and dust sensing to obtain a detailed picture of Pluto, its composition both on the surface and its atmosphere, its moons and its environment.
These are New Horizons’ instruments:
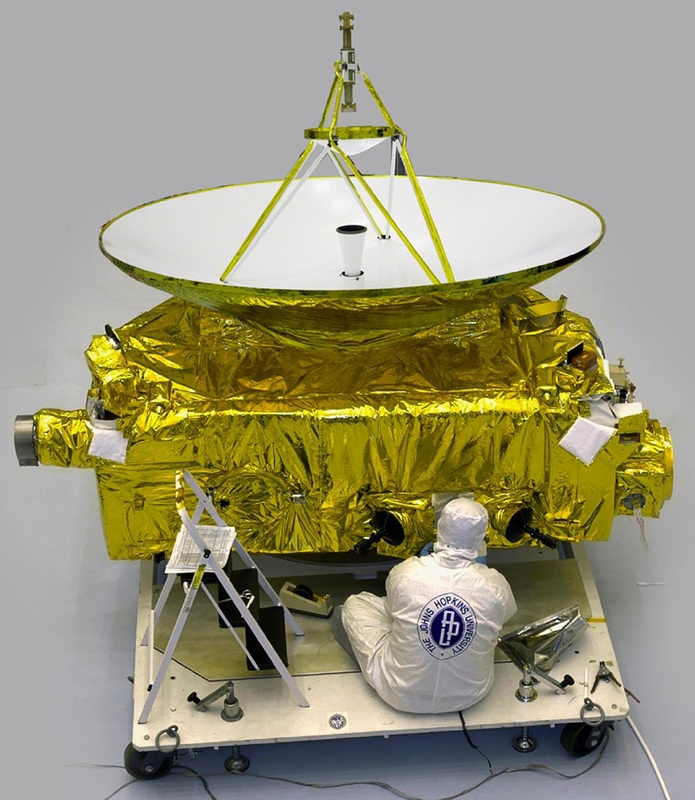
[Alice and Ralph are also collectively referred to as PERSI – Pluto Exploration Remote Sensing Investigation.]
The fundamental (Group 1) science objectives of the New Horizons mission can be achieved with the core science payload comprised of Alice, REX and Ralph.
The supplemental payload deepens and broadens the mission science, but is not required to achieve the minimum criteria for a mission success. The boresights of the Ralph, LORRI, and Alice airglow channel are aligned with the spacecraft –X axis allowing them to operate simultaneously.